Newton’s Laws of Motion
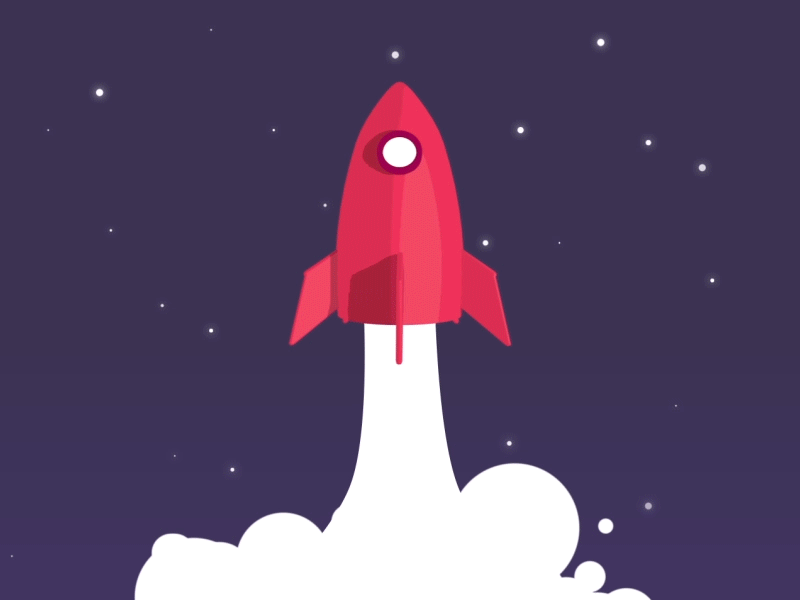
Sir Isaac Newton’s Laws of Motion, formulated in the 17th century, remain monumental pillars in classical mechanics, providing profound insights into the principles governing the motion of objects. This exploration delves into each of Newton’s three laws, unraveling their significance, implications, and real-world applications.
Newton’s First Law: The Law of Inertia
Definition: Newton’s First Law states that an object at rest will remain at rest, and an object in motion will continue moving with a constant velocity unless acted upon by an external force. This principle encapsulates the concept of inertia, the tendency of an object to resist changes in its state of motion.
Implications: The Law of Inertia lays the groundwork for understanding how objects behave in the absence of external influences. It clarifies why a stationary car remains at rest until an external force, such as an engine or a push, overcomes its inertia and sets it in motion.
Newton’s Second Law – The Law of Acceleration:
2.1 Definition: Newton’s Second Law introduces the relationship between force, mass, and acceleration. It states that the acceleration of an object is directly proportional to the net force acting upon it and inversely proportional to its mass.
2.2 Implications: This law unveils the dynamics of force application and its impact on an object’s motion. It explains why a larger force is required to accelerate a massive object and why a smaller force can produce significant acceleration in a less massive one. It is a fundamental equation in understanding the interaction between forces and motion.
Newton’s Third Law: The Action-Reaction Principle
Newton’s Third Law states that for every action, there is an equal and opposite reaction. This means that any force exerted by one object on another is met with an equal force in the opposite direction exerted by the second object on the first. The forces always occur in pairs, simultaneously and in opposite directions.
Implications: This law unveils the concept that forces are interactions between pairs of objects. It explains phenomena such as propulsion in rockets, where the expulsion of gases downward generates an upward reaction force, propelling the rocket forward. Understanding action and reaction pairs is crucial to comprehending various mechanical systems.
Real-world Applications:
Automotive Safety: Newton’s laws find applications in automotive safety, particularly in the design of airbags. During a collision, a passenger’s body tends to continue moving due to inertia. The airbag deploys, exerting a force that reduces the passenger’s deceleration and lessens the impact force.
Space Exploration: The laws of motion are fundamental in space exploration. Newton’s Third Law, the action-reaction principle, is central to rocket propulsion. As exhaust gases are expelled downward, the rocket experiences an equal and opposite force propelling it upward.
Sports Dynamics: Newton’s laws play a crucial role in sports dynamics. The techniques used in throwing, hitting, or kicking objects involve an understanding of force, mass, and acceleration. Athletes leverage these principles to optimize their performance.
Limitations and Considerations:
Friction and Other Forces: Newton’s laws assume idealized conditions without factors such as friction, air resistance, or other external forces. In real-world scenarios, these factors can significantly impact the behavior of objects in motion.
Relativity and High Speeds: Newton’s laws are accurate for objects moving at everyday speeds, but they become less applicable in the realm of high speeds or extreme gravitational fields. In these cases, Einstein’s theory of relativity takes precedence.
Integration with Other Physics Principles:
Kinematics: Newton’s laws seamlessly integrate with kinematics, the study of motion without considering the forces causing it. Together, they provide a comprehensive framework for understanding the complete dynamics of motion.
Energy Conservation: The laws of motion intertwine with the principles of energy conservation. Forces doing work on objects, as described by Newton’s laws, lead to changes in kinetic and potential energy.
Challenges and Innovations:
Non-uniform Motion: Newton’s laws work best when describing uniform motion or simple scenarios. Describing non-uniform motion or intricate systems may necessitate advanced mathematical techniques or alternative physics models.
Innovations in Aerospace: Innovations in aerospace engineering continually push the boundaries of Newton’s laws. Space exploration, for instance, requires a delicate balance of forces, trajectories, and gravitational influences, demanding innovative solutions beyond classical mechanics.
Newton’s Laws of Motion are one of the most important ideas in physics. They give us a solid way to understand how motion works. These laws affect many parts of our physical world, from the simple Law of Inertia to the complex Law of Acceleration and the Action-Reaction Principle, which explain how forces combine.
Newton’s ideas had a huge effect on our understanding of the basic rules of motion, as shown by the fact that they are still used and relevant in many fields, from engineering to sports science.
Simple Machines
Simple machines, the foundational elements of mechanical systems, are ingenious devices that amplify or redirect force, making tasks more manageable. This exploration delves into the six classical simple machines, exploring their definitions, mechanisms, and the pivotal roles they play in everyday life and complex machinery.
Lever:
A lever is a rigid rod or beam pivoted at a fixed point called the fulcrum. It consists of three key components: the effort force (applied force), the load (resistance force), and the fulcrum. Levers are categorized into three classes based on the relative positions of these components.
Mechanism: Levers operate on the principle of torque, where the force applied at one end of the lever generates rotational motion around the fulcrum, allowing the load to be lifted or moved with less effort.
Real-world Examples: Examples of levers abound in everyday life, such as seesaws, scissors, and crowbars. In machinery, levers are prevalent in devices like bottle openers and hand trucks.
Wheel and Axle:
The wheel and axle consist of a large wheel (the wheel) and a smaller rod or axle positioned at its center. This simple machine enables the transfer of force from the applied effort to the load, producing rotational motion.
Mechanism: Rotation occurs as a result of the force applied to the wheel or axle. The mechanical advantage is determined by the ratio of the wheel’s radius to the axle’s radius.
Real-world Examples: Common examples include wheels on cars, bicycles, and doorknobs. In machinery, the wheel and axle configuration is found in items like hand-cranked winches and waterwheels.
Pulley:
Definition: A pulley is a grooved wheel mounted on an axle or shaft with a rope or cable running in the groove. Pulleys are classified as fixed, movable, or compound, depending on their configurations.
Mechanism: Pulleys facilitate the redirection of force and allow for the lifting or lowering of loads with less effort. Movable pulleys increase the mechanical advantage, while fixed pulleys change the direction of the force.
Real-world Examples: Elevators, flagpoles, and blinds often utilize pulley systems. In industrial settings, pulleys are integral components of conveyor belts and cranes.
Inclined Plane:
An inclined plane is a flat surface set at an angle to the horizontal. It provides a gradual slope for an object to be raised or lowered.
Mechanism: Inclined planes reduce the amount of force needed to move an object vertically by increasing the distance over which the force is applied.
Real-world Examples: Ramps, stairs, and sloping roads are common examples of inclined planes. In machinery, conveyor belts and chutes utilize the principle of inclined planes for material transport.
Wedge:
A wedge is a triangular-shaped device with a thin edge that tapers to a thicker base. It functions by splitting or separating objects.
Mechanism: The force applied to the wedge translates into a splitting force, allowing it to penetrate and separate materials. Wedges are versatile tools used for cutting, lifting, or holding objects in place.
Real-world Examples: Examples of wedges include knives, axes, and chisels. In construction, wedges are employed in shims and doorstops.
Screw:
Definition: A screw is an inclined plane wrapped around a central axis in a helical shape. It consists of a threaded rod or cylinder and functions as both a rotational and linear force device.
Mechanism: When the screw is rotated, it moves along its axis, providing a combination of rotational and linear motion. This allows for controlled fastening, lifting, or compression.
Real-world Examples: Screws are ubiquitous in construction, manufacturing, and everyday items such as bolts, nuts, and jar lids. They are also integral in tools like screwdrivers.
Applications and Importance:
Mechanical Advantage: Simple machines enhance mechanical advantage, making it possible to perform tasks with less effort. This efficiency is crucial in various fields, from construction and manufacturing to transportation and household chores.
Compound Machines: Many complex machines are combinations of multiple simple machines working together. This synergy enhances the overall efficiency and functionality of intricate systems.
Limitations and Considerations:
Friction and Energy Loss: Simple machines are not entirely efficient, and factors like friction result in energy losses. Real-world applications often require considerations for these losses.
Mechanical Advantage vs. Distance: Simple machines operate on the principle of mechanical advantage, but this may come at the cost of increased distance. It’s essential to